Possible Adverse Effects of Food Additive E171 (Titanium Dioxide) Related to Particle Specific Human Toxicity, Including the Immune System
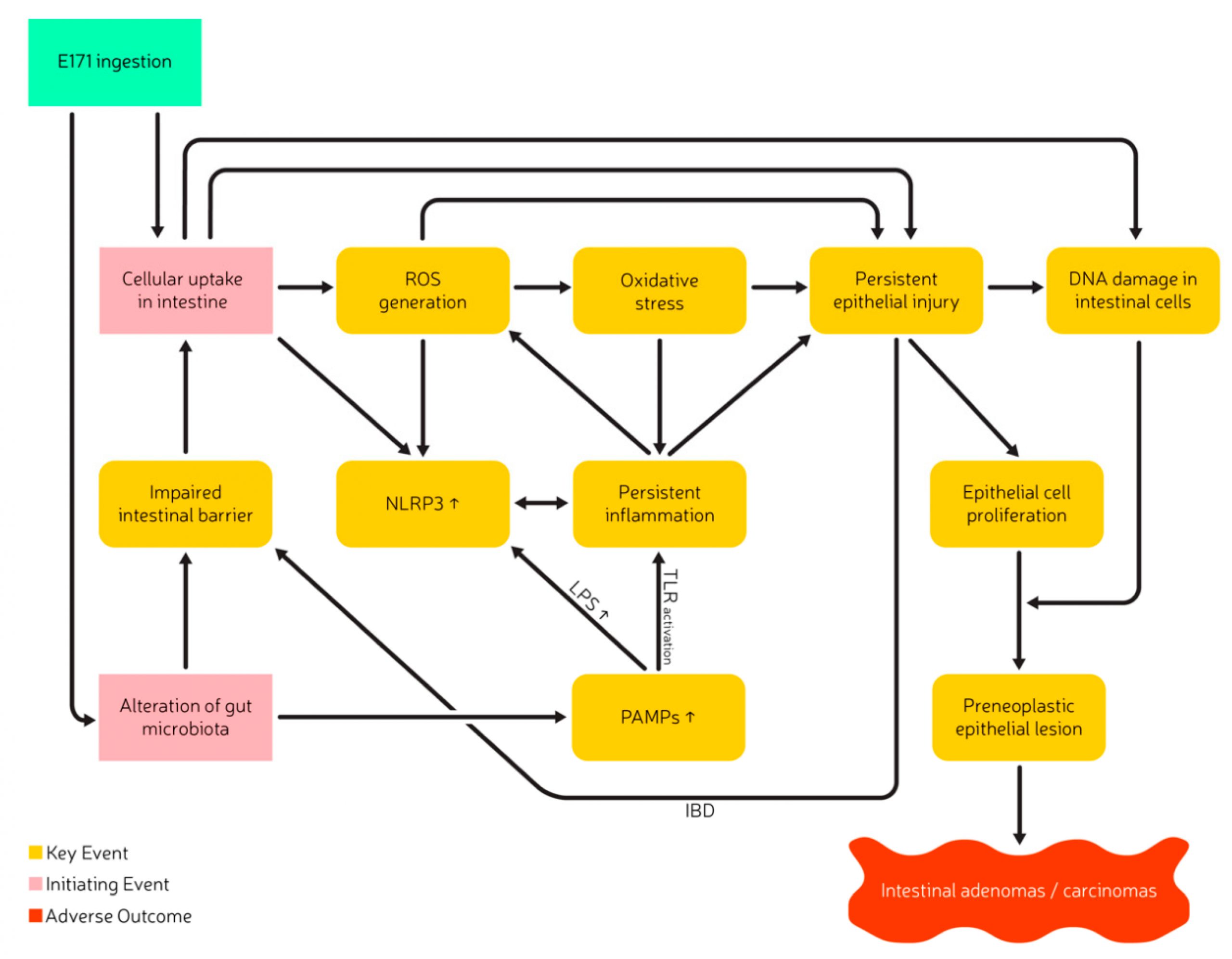
In 2019, France made the decision to completely ban TiO2 in the nutraceutical sector from January 1, 2020. Now, the EFSA panel concluded, that E171 (TiO2) can no longer be considered as safe when used as a food additive in Europe on the basis of the following article. Read more about the possible consequences in the pharmaceutical industry in our special article about TiO2 here!
Titanium dioxide (TiO2) is used as a food additive (E171) and can be found in sauces, icings, and chewing gums, as well as in personal care products such as toothpaste and pharmaceutical tablets. Along with the ubiquitous presence of TiO2 and recent insights into its potentially hazardous properties, there are concerns about its application in commercially available products. Especially the nano-sized particle fraction (<100 nm) of TiO2 warrants a more detailed evaluation of potential adverse health effects after ingestion.
A workshop organized by the Dutch Office for Risk Assessment and Research (BuRO) identified uncertainties and knowledge gaps regarding the gastrointestinal absorption of TiO2, its distribution, the potential for accumulation, and induction of adverse health effects such as inflammation, DNA damage, and tumor promotion. This review aims to identify and evaluate recent toxicological studies on food-grade TiO2 and nano-sized TiO2 in ex-vivo, in-vitro, and in-vivo experiments along the gastrointestinal route, and to postulate an Adverse Outcome Pathway (AOP) following ingestion. Additionally, this review summarizes recommendations and outcomes of the expert meeting held by the BuRO in 2018, in order to contribute to the hazard identification and risk assessment process of ingested TiO2.
Download the full article as a PDF here or read it here
Background of TiO2 as a Food Additive
-
Does oral exposure to E171 or nano-sized TiO2 reveal a relevant toxicological hazard?
-
How reliable are these in-vitro and in-vivo studies?
-
Are the animal models, the exposure conditions, and the effects observed in these studies relevant to humans?
-
Can the data from in-vitro and in-vivo studies with TiO2 be extrapolated to humans?
-
Are there epidemiological studies on the effects of E171 in humans after oral exposure?
Since this workshop in 2018, more studies have been published that investigated the concerns of adverse effects arising from E171 ingestion. This literature review integrates the main conclusions of the expert meeting initiated by BuRO, with recently published studies in order to present an overview of relevant findings regarding E171 toxicity after oral intake. The literature search on PubMed and EmBase was conducted from June 2020–September 2020 and included the search criteria “TiO2”, “titanium dioxide”, “E171” with publication dates from 2018–2020. Previous scientific papers in the field, as well as references in these publications were evaluated. The present literature review aims to shed light on the importance of complete particle characterization, on the effects of matrices, and highlight toxicological relevant pathways potentially involved in the induction of adverse health effects following E171 ingestion. Additionally, it provides approaches to decrease uncertainties concerning the health effects of E171 consumption, and finally formulates recommendations for future studies and follow-up actions regarding the risk assessment of E171.
Physicochemical Properties and Characterization of E171

For this reason, it is important to carefully examine and analyze the physicochemical characteristics of TiO2 particles in its vehicle, as well as in its surrounding matrix as their final milieu, to guarantee a profound assessment of potential adverse health effects of E171 and to adequately compare different studies in the process of risk assessment.
Exposure to E171
E171 is used in products such as candy, coffee creamer, chewing gum, sauces, nutritional supplements, toothpaste, and pharmaceuticals. Although both the anatase and rutile forms of TiO2 are authorized for foods, the characterization of European and American food samples showed that anatase is the predominant TiO2 crystalline structure used as food additive E171 and thus the main source of exposure for the general population [27,45,46,47,48]. The intake of E171 varies between different age groups and countries, while children, in general are the most highly exposed group, due to their lower body mass and disproportionally higher consumption of E171-containing products [5,49]. Table 1 shows the estimated daily intake of E171 per kg body weight (bw) in different countries and age-groups [49,50].
Table 1. Mean and 95th percentile estimation of daily oral intake of TiO2 from food products (E171), food supplements and toothpaste in different age groups and countries in mg/kg bw/day (n/a = data not available, * mg/person/day).
Author | Year | Country | Mean (mg/kg bw/Day) | 95th Percentile (mg/kg bw/Day) |
---|---|---|---|---|
Wu [50] | 2020 | USA | 0.15-3.9 * (PCP survey and usage patterns, no food included) | n/a |
EFSA [21] | 2016 | Europe | <11 months: 0.2-0.8 | <11 months: 0.7-3.9 |
1-3 years: 0.6-4.6 | 1-3 years: 2.0-6.8 | |||
3-9 years: 0.9-5.5 | 3-9 years: 2.4-14.8 | |||
10-17 years: 0.4-4.1 | 10-17 years: 1.3-10.8 | |||
18-64 years: 0.3-4.0 | 18-64 years: 1.1-9.7 | |||
>65 years: 0.2-2.8 | >65 years: 0.5-7.0 | |||
Rompelberg [49] | 2016 | NL | 2-6 years: 0.66-0.70 | 2-6 years: 1.19-1.40 |
7-69 years: 0.16-0.18 | 7-69 years: 0.47-0.54 | |||
>69 years: 0.05-0.07 | >69 years: 0.20-0.28 | |||
Bachler [51] | 2015 | DE | "Other Children": ~2 | "Other Children": ~0.7-7.2 |
Toddlers, adolescents, adults, elderly: 0.5-1 | Toddlers, adolescents, adults, elderly: ~0.1-4.2 | |||
Sprong [52] | 2015 | NL | 2-6 years: 1.3-1.5 | 2-6 years: 4.5-5.6 |
7-69 years: 0.6-0.7 | 7-69 years: 2.6-3.0 | |||
>70 years: 0.5-0.6 | >70 years: 1.7-2.2 | |||
Christensen [53] | 2015 | DK | Children: 2 | n/a |
Adults: 1 | ||||
Weir [27] | 2012 | UK | <10 years 2-3 | n/a |
>10 years: 1 | ||||
Weir [27] | 2012 | US | <10 years: 1-2 | n/a |
>10 years: 0.2-0.7 | ||||
Powell [54] | 2010 | UK | 5 * | n/a |
The highest concentrations of E171 are found in chewing gum, candies, and powder sugar toppings such as icings. Chewing gums contain between 1.1 mg (±0.3 mg) to 17.3 mg (±0.9 mg) TiO2 particles per piece of gum with a mean average weight per piece of 1416 mg (±27 mg) to 2240 mg (±86 mg) [26]. TiO2 nanoparticles account for up to 19% (±4) of all particles present in these gums [26]. The accidental ingestion of toothpaste, while brushing teeth is another major source of E171 intake, that can result in an exposure of 0.15 to 3.9 mg/day, when 10% of toothpaste is ingested [50]. Additional release of TiO2 particles (70–200 nm) from food packaging materials or food-related products, such as frying pans, may also contribute to TiO2 ingestion [55]. The focus of oral TiO2 exposure estimation should potentially be extended from the food additive E171 to personal care products, packaging, and coating of household items [28,33,55]. Daily dietary intake of E171 can reach several hundred milligrams, of which at least 10–40% are in the form of TiO2 nanoparticles. The long-term exposure to such quantities of nano- and micro-sized TiO2 raises concerns about the risk of potential accumulation in organs and potentially harmful effects on human health [27].
Article information: Bischoff, N.S.; de Kok, T.M.; Sijm, D.T.H.M.; van Breda, S.G.; Briedé, J.J.; Castenmiller, J.J.M.; Opperhuizen, A.; Chirino, Y.I.; Dirven, H.; Gott, D.; Houdeau, E.; Oomen, A.G.; Poulsen, M.; Rogler, G.; van Loveren, H. Possible Adverse Effects of Food Additive E171 (Titanium Dioxide) Related to Particle Specific Human Toxicity, Including the Immune System. Int. J. Mol. Sci. 2021, 22, 207. https://doi.org/10.3390/ijms22010207